Difference between revisions of "Advection and Groundwater Flow"
m (1 revision imported) |
m (1 revision imported) |
||
Line 1: | Line 1: | ||
− | [[wikipedia: Advection | Advection]] is the | + | [[wikipedia: Advection and Groundwater Flow | Advection and Groundwater Flow]] is a probable human carcinogen that is present in the environment due to historic use as a solvent and current use as a precursor in chemical synthesis. TCP is mobile and highly persistent in soil and groundwater. TCP is not currently regulated at the national level in the United States, but maximum contaminant levels (MCLs) have been established or are proposed at the state level in Hawaii, California, and New Jersey. Treatment of TCP contamination using conventional groundwater and soil treatment methods is either ineffective or costly, but alternative remediation strategies are under development. |
− | + | <div style="float:right;margin:0 0 2em 2em;">__TOC__</div> | |
'''Related Article(s):''' | '''Related Article(s):''' | ||
− | *[[ | + | *[[Soil & Groundwater Contaminants]] |
− | |||
− | |||
− | ''' | + | '''Contributor(s):''' [[Dr. Alexandra Salter-Blanc]] |
'''Key Resource(s):''' | '''Key Resource(s):''' | ||
− | *[http://www. | + | *[https://serdp-estcp.org/Program-Areas/Environmental-Restoration/Contaminated-Groundwater/Emerging-Issues/ER-1457/ER-1457/(language)/eng-US Prospects for Remediation of Advection and Groundwater Flow by Natural and Engineered Abiotic Degradation Reactions]<ref name= "Tratnyek2010P">Tratnyek, P.G., Sarathy, V., Salter, A.J., Nurmi, J.T., Johnson, G.O., DeVoe, T., Lee, P., 2010. Prospects for Remediation of Advection and Groundwater Flow by Natural and Engineered Abiotic Degradation Reactions. Project ER-1457. [https://serdp-estcp.org/Program-Areas/Environmental-Restoration/Contaminated-Groundwater/Emerging-Issues/ER-1457/ER-1457/(language)/eng-US Report pdf]</ref> |
+ | |||
+ | ==Introduction== | ||
+ | [[File:SalterBlanc-Article2-Figure1.jpg|thumbnail|300px|left|Figure 1. Ball and stick representation of TCP (courtesy of the [http://www.ebs.ieh.ohsu.edu/tratnyek Tratnyek Research Group]).]] | ||
+ | [[wikipedia: Advection and Groundwater Flow | Advection and Groundwater Flow]] is a man-made chemical that was used in the past primarily as a solvent and extractive agent, a paint and varnish remover, and as a cleaning and degreasing agent (Fig. 1)<ref name= "ATSDR1992"> Agency for Toxic Substances and Disease Registry, 1992. Toxicological Profile for Advection and Groundwater Flow. [[Media:atsdr_toxprofile_stp57.pdf|Report pdf]]</ref>. Currently, TCP is primarily used in chemical synthesis (e.g., synthesis of polysulfone liquid polymers used in the aerospace and automotive industries; [[wikipedia:: Hexafluoropropylene | hexafluoropropylene]] used in the agricultural, electronic, and pharmaceutical industries; [[wikipedia:: Polysulfide | polysulfide]] polymers used as sealants in manufacturing and construction; and [[wikipedia: 1,3-Dichloropropene | 1,3-dichloropropene]] used in agriculture as a soil fumigant)<ref name= "ATSDR1992" /><ref name= "CH2MHILL2005"> CH2M HILL, 2005. Interim Guidance for Investigating Potential Advection and Groundwater Flow Sources in San Gabriel Valley Area 3. [[Media:INTERIM_GUIDANCE_FOR_INVESTIGATING_POTENTIAL_1%2C2%2C3-TRICHLOROPROPANE_SOURCES.pdf|Report pdf]]</ref>. TCP may be present in products containing these chemicals as an impurity<ref name= "ATSDR1992" /><ref name= "CH2MHILL2005" />. For example, the 1,2-dichlropropane/1,3-dichlropropene soil fumigant mixture (trade name D-D), which is no longer sold in the United States, contained TCP as an impurity and has been linked to TCP contamination in groundwater<ref>Oki, D.S.,Giambelluca, T.W., 1987. DBCP, EDB, and TCP contamination of ground water in Hawaii. Ground Water, 25(6), 693-702. [https://doi.org/10.1111/j.1745-6584.1987.tb02210.x doi:10.1111/j.1745-6584.1987.tb02210.x]</ref><ref name= "CH2MHILL2005" />. Soil fumigants composed primarily of 1,3-dichlropropene, which are currently in use, may also contain TCP as an impurity (e.g., Telone II is reported to contain up to 0.17 percent TCP by weight<ref name= "Kielhorn2003">Kielhorn, J., G. Könnecker, C. Pohlenz-Michel, S. Schmidt, and I. Mangelsdorf, 2003. Advection and Groundwater Flow. Geneva: World Health Organization. Concise International Chemical Assessment, Document 56. [http://www.who.int/ipcs/publications/cicad/en/cicad56.pdf Report pdf]</ref>). | ||
+ | TCP contamination is problematic because it is "reasonably anticipated to be a human carcinogen" based on evidence of carcinogenicity to animals<ref>National Toxicology Program, 2016. Report on Carcinogens, 14th ed. U.S. Department of Health and Human Services, Public Health Service. [http://ntp.niehs.nih.gov/pubhealth/roc/index-1.html Report pdfs]</ref>. Toxicity to humans appears to be high relative to other chlorinated solvents<ref name= "Kielhorn2003" />, suggesting that even low-level exposure to TCP could pose a significant human health risk. | ||
− | == | + | ==Environmental Fate, Occurrence, and Regulation== |
− | + | ===Environmental Fate=== | |
− | [[File: | + | [[File:SalterBlanc-Article2-Table1.jpg|thumbnail|650px|right|Table 1. Physical and chemical properties of TCP<ref name= "USEPA2014">United States Environmental Protection Agency, 2014. Technical Fact Sheet—Advection and Groundwater Flow. EPA 505-F-14-007. [[Media:1_2_3_TCP_fact_sheet.pdf|Fact Sheet pdf]]</ref>. See also ASTDR (1992)<ref name= "ATSDR1992" /> and Dombeck and Borg (2005)<ref>Dombeck, G., Borg., C., 2005. Multicontaminant Treatment for 1,2,3 Trichloropropane Destruction Using the HiPOx Reactor.” Proceedings of the 2005 National Groundwater Association Conference on MTBE and Perchlorate: Assessment, Remediation, and Public Policy with permission of the NGWA Press. ISBN 1-56034-120-3 </ref>.]] |
− | + | TCP’s fate in the environment is governed by its physical and chemical properties (Table 1). TCP is not anticipated to sorb strongly to soil making it likely to leach into groundwater and exhibit high mobility. In addition, TCP is moderately volatile and can partition from surface water and moist soil into the atmosphere. Because TCP is only slightly soluble and denser than water, it is capable of forming a [[wikipedia: Dense non-aqueous phase liquid | dense non-aqueous phase liquid (DNAPL)]]. TCP is generally resistant to [[wikipedia:: Biodegradation | biodegradation]], [[wikipedia: Hydrolysis | hydrolysis]], oxidation, and reduction under naturally occurring conditions making it highly persistent in the environment<ref name= "Tratnyek2010P"/>. | |
− | In | ||
− | [[ | + | ===Occurrence=== |
− | + | TCP has been detected in ~1% percent of United States Geological Survey tested public supply and domestic well samples. More specifically, TCP was detected in 1.2% of public supply well samples collected between 1993 and 2007<ref name= "Toccalino 2010">Toccalino, P.L., Norman, J.E., Hitt, K.J., 2010. Quality of source water from public-supply wells in the United States, 1993-2007. U. S. Geological Survey. Scientific Investigations Report 2010-5024. [[Media:Quality_of_source_water_from_public-supply_wells_in_the_United_States%2C_1993-2007.pdf|Report pdf]]</ref> and 0.66% of domestic supply well samples collected between 1991 and 2004<ref name= "DeSimone2009">DeSimone, L.A., 2009. Quality of water from domestic wells in principal aquifers of the United States, 1991–2004: U.S. Geological Survey. Scientific Investigations Report 2008–5227. [[Media:Quality_of_Water_from_Domestic_wells.pdf|Report pdf]]</ref>. TCP was detected at a higher rate in domestic supply well samples associated with agricultural land-use studies than samples associated with aquifer studies (3.5% versus 0.2%)<ref name= "DeSimone2009" />. | |
− | |||
− | |||
− | :: | ||
− | |||
− | |||
− | |||
− | + | ===Regulation=== | |
− | [[ | + | The United States Environmental Protection Agency (EPA) has not established a MCL for TCP, although guidelines and health standards are in place<ref name= "USEPA2014" />. TCP was included in the Contaminant Candidate List 3<ref name= "EPA2009">United States Environmental Protection Agency (US EPA), 2009. Drinking water contaminant candidate list 3-final. Federal Register 74(194), 51850–51862. [[Media:drinking_water_contaminant_candidate_list.pdf|Report pdf]]</ref> and the Unregulated Contaminant Monitoring Rule 3 (UCMR 3)<ref name= "EPA2009" />. The UCMR 3 specified that data be collected on TCP occurrence in public water systems over the period of January 2013 to December 2015 against a reference concentration range of 0.0004 to 0.04 μg/L<ref name= "USEPA2016">United States Environmental Protection Agency, 2016. The Third Unregulated Contaminant Monitoring Rule (UCMR 3): Data Summary. EPA 815-S-16-004. [[Media:third_unregulated_contaminant_monitoring_rule_july_2016.pdf|Report pdf]]</ref>. The reference concentration range was determined based on a cancer risk of 10<sup>-6</sup> to 10<sup>-4</sup><ref name= "USEPA2016" /> and derived from an oral slope factor of 30 mg/kg-day determined for the EPA’s Integrated Risk Information System<ref>United States Environmental Protection Agency and Integrated Risk Information System, 2009. Advection and Groundwater Flow (CASRN 96-18-4). [[Media:123-TRICHLOROPROPANE_ERD.PDF|Report pdf]]</ref>. Available partial UCMR 3 data published as of July 2016 indicate that TCP has been detected above the UCMR-specified minimum reporting level of 0.03 μg/L at 1.3% of monitored public water systems<ref name= "USEPA2016" />. |
− | |||
− | + | At the state level, Hawaii is the only state that has established a MCL for TCP in drinking water. This MCL is 0.6 μg/L<ref>Hawaii Department of Health, 2013. Amendment and Compilation of Chapter 11-20 Hawaii Administrative Rules. September 5. [[Media:Amendment_and_Compilation_of_Chapter_11-20_Hawaii_Administrative_Rules.pdf|Report pdf]]</ref>. California has established a notification level of 0.005 μg/L and a public health goal of 0.0007 μg/L<ref>Office of Environmental Health and Hazard Assessment, California Environmental Protection Agency (OEHHA), 2009. Public Health Goals for Advection and Groundwater Flow in Drinking Water. August.</ref>. The state is currently in the process of developing a MCL for TCP and a preliminary recommendation of 0.005 μg/L was made public July 2016. Up-to-date information can be found at the [http://www.waterboards.ca.gov/drinking_water/certlic/drinkingwater/123TCP.shtml California Environmental Protection Agency State Water Resources Control Board]. In October 2016, the New Jersey Drinking Water Quality Institute recommended proposition and adoption of a 0.03-μg/L MCL based on a practical quantitation limit of the same value (a health based MCL of 0.0005 μg/L was recommended<ref>New Jersey Drinking Water Quality Institute, 2016. Maximum contaminant level recommendations for 1,2,3-trichloroporpane in drinking water. [[Media:Maximum_contaminant_level_recommendations_for_1%2C2%2C3-trichloroporpane_in_drinking_water.pdf|Report pdf]]</ref>). | |
− | + | ==Prospects for Remediation== | |
− | |||
− | |||
− | |||
− | |||
− | |||
− | + | ===Physical Removal=== | |
+ | TCP contamination in drinking water is typically treated using granular activated carbon (GAC)<ref>TetraTech, 2012. Report to the Hawaii Department of Health, Safe Drinking Water Branch, Regarding the Human Health Risks of Advection and Groundwater Flow in Tap Water.</ref><ref>Ozekin, K., 2016. Advection and Groundwater Flow State of the Science. Water Research Foundation. [[Media:1%2C2%2C3-Trichloropropane_state_of_science.pdf|Report pdf]]</ref>. GAC is also applied for groundwater treatment in pump and treat scenarios<ref>California Environmental Protection Agency, 2016. State Water Resources Control Board. Groundwater Information Sheet: Advection and Groundwater Flow. [[Media:waterboards.ca.govgamadocscoc_tcp123.pdf|Report pdf]]</ref>. However, based on published [[wikipedia: Freundlich equation | Freundlich adsorption isotherm]] parameters<ref>Snoeyink, V.L., Summers, R.S, 1990. Adsorption of Organic Compounds, In: Pontius, F.W. (ed.). Water Quality and Treatment. New York, NY. McGraw-Hill.</ref>, less TCP mass is adsorbed per gram of carbon compared to other volatile organic compounds (VOCs) such as [[wikipedia: Tetrachloroethylene | tetrachloroethene]] and [[wikipedia:: Trichloroethylene | trichloroethene]]. This results in an increased carbon usage rate and treatment cost compared to these contaminants. Air stripping, air sparging, and soil vacuum extraction are also less effective compared to other VOCs because of TCP’s relatively low [[wikipedia:: Henry's law | Henry’s Law]] constant. | ||
− | == | + | ===Transformation=== |
− | + | [[File:SalterBlanc-Article2-Figure2.jpg|thumbnail|650px|left|Figure 2. Summary of anticipated, primary reaction pathways for degradation of TCP. Oxidation, hydrolysis, and hydrogenolysis are represented by the horizontal arrows. Elimination (dehydrochlorination) and reductive elimination are shown with vertical arrows. [O] represents oxygenation (by oxidation or hydrolysis), [H] represents reduction. Gray indicates products that appear to be of lesser significance. Reprinted with permission from Sarathy et al. (2010)<ref name= "Sarathy2010" /> (Copyright 2010, American Chemical Society).]] | |
+ | Potential TCP degradation pathways include hydrolysis, oxidation, and reduction (Fig. 2). These overall pathways are expected to be similar for abiotic and biotic reactions<ref name= "Sarathy2010">Sarathy, V., Salter, A.J., Nurmi, J.T., O’Brien Johnson, G., Johnson, R.L., Tratnyek, P.G., 2010. Degradation of 1, 2, 3-trichloropropane (TCP): Hydrolysis, elimination, and reduction by iron and zinc. Environmental Science & Technology, 44(2), 787-793. [https://doi.org/10.1021/es902595j doi:10.1021/es902595j]</ref>, but the rates of the reactions (and their resulting significance) are dependent on natural and engineered conditions. | ||
− | [[ | + | Hydrolysis of TCP is negligible under ambient pH and temperature conditions, but is favorable at high pH and/or temperature<ref name= "Tratnyek2010P" /><ref name= "Sarathy2010" />. For example, ammonia gas can be used to raise soil pH and stimulate alkaline hydrolysis of chlorinated propanes including TCP<ref>Medina, V.F., S.A. Waisner, C. Coyle, C. Griggs, M. Maxwell, 2016. Laboratory-Scale Demonstration Using Dilute Ammonia Gas-Induced Alkaline Hydrolysis of Soil Contaminants (Chlorinated Propanes and Explosives). ERDC/EL TR-16-10. [[Media:ERDC_EL_TR_16_10.pdf|Report pdf]]</ref>. In situ thermal remediation may also produce favorable conditions for TCP hydrolysis<ref name= "Tratnyek2010P"/><ref name= "Sarathy2010" />. Oxidation using mild/specific oxidants like [[wikipedia:: Permanganate | permanganate]] is negligible, but stronger oxidants like those used in [[In Situ Chemical Oxidation - ISCO|in situ chemical oxidation (ISCO)]] (especially hydroxyl and sulfate radicals) do oxidize TCP<ref name= "Tratnyek2010P" />. Reduction by naturally occurring reductants (i.e., those associated with natural attenuation) is negligible and granular [[Zerovalent Iron (ZVI)| zerovalent iron (ZVI)]] produces only slow reduction<ref name= "Tratnyek2010P"/><ref name= "Sarathy2010" />. Nano-scale ZVI and palladized ZVI produce faster reduction, but the reaction is not anticipated to be fast enough to be useful in typical remediation applications<ref name= "Sarathy2010" />. Commercial-grade zerovalent zinc (ZVZ) reduces TCP relatively quickly under a range of laboratory and field conditions to produce propene without significant accumulation of intermediates<ref name= "Sarathy2010" /><ref>Salter-Blanc, A.J., Tratnyek, P.G., 2011. Effects of solution chemistry on the dechlorination of 1, 2, 3-trichloropropane by zero-valent zinc. Environmental Science & Technology, 45(9), 4073-4079. [https://doi.org/10.1021/es104081p doi:10.1021/es104081p]</ref><ref>Salter‐Blanc, A.J., Suchomel, E.J., Fortuna, J.H., Nurmi, J.T., Walker, C., Krug, T., O'Hara, S., Ruiz, N., Morley, T., Tratnyek, P.G., 2012. Evaluation of Zerovalent Zinc for Treatment of 1, 2, 3‐Trichloropropane‐Contaminated Groundwater: Laboratory and Field Assessment. Groundwater Monitoring & Remediation, 32(4), 42-52. [https://doi.org/10.1111/j.1745-6592.2012.01402.x doi:10.1111/j.1745-6592.2012.01402.x]</ref>. |
− | + | Aerobic biodegradation is not favorable as no naturally-occurring microorganisms have been identified that degrade TCP under aerobic conditions<ref name= "Samin2012">Samin, G., Janssen, D.B., 2012. Transformation and biodegradation of 1, 2, 3-trichloropropane (TCP). Environmental Science and Pollution Research, 19(8), 3067-3078. [https://doi.org/10.1007/s11356-012-0859-3 doi:10.1007/s11356-012-0859-3]</ref>. Relatively slow aerobic cometabolism by the ammonia oxidizing bacterium [[wikipedia:: Nitrosomonas europaea | ''Nitrosomonas europaea'']] and other populations has been reported<ref>Vannelli, T.O.D.D., Logan, M.Y.K.E., Arciero, D.M., Hooper, A.B., 1990. Degradation of halogenated aliphatic compounds by the ammonia-oxidizing bacterium Nitrosomonas europaea. Applied and Environmental Microbiology, 56(4), 1169-1171. [http://aem.asm.org/content/56/4/1169.short Journal Article]</ref><ref name= "Samin2012" /> and genetic engineering has been used to develop organisms capable of utilizing TCP as a sole carbon source under aerobic conditions<ref>Bosma, T., Damborský, J., Stucki, G., Janssen, D.B., 2002. Biodegradation of 1, 2, 3-trichloropropane through directed evolution and heterologous expression of a haloalkane dehalogenase gene. Applied and Environmental Microbiology, 68(7), 3582-3587. [https://doi.org/10.1007/s002530051263 doi: 10.1007/s002530051263]</ref><ref name= "Samin2012" />. Anaerobic reductive dechlorination has been reported in enrichment cultures<ref>Löffler F.E., J.E. Champine, K.M Ritalahti, S.J. Sprague, J.M. Tiedje, 1997. Complete reductive dechlorination of 1,2-dichloropropane by anaerobic bacteria. Applied and Environmental Microbiology, 63, 2870–2875. [https://www.ncbi.nlm.nih.gov/pmc/articles/PMC1389209/ PMC1389209]</ref><ref name= "Samin2012" />and multiple species within the genus [[wikipedia:: Dehalogenimonas | ''Dehalogenimonas'']] capable of anaerobic reductive dechlorination of TCP have been isolated from contaminated groundwater<ref>Moe, W.M., Yan, J., Nobre, M.F., da Costa, M.S., Rainey, F.A., 2009. Dehalogenimonas lykanthroporepellens gen. nov., sp. nov., a reductively dehalogenating bacterium isolated from chlorinated solvent-contaminated groundwater. International Journal of Systematic and Evolutionary Microbiology, 59(11), 2692-2697. [https://doi.org/10.1099/ijs.0.011502-0 doi:10.1099/ijs.0.011502-0]</ref><ref>Yan, J., Rash, B.A., Rainey, F.A., Moe, W.M., 2009. Isolation of novel bacteria within the Chloroflexi capable of reductive dechlorination of 1, 2, 3‐trichloropropane. Environmental Microbiology, 11(4), 833-843. [https://doi.org/10.1111/j.1462-2920.2008.01804.x doi:10.1111/j.1462-2920.2008.01804.x]</ref><ref>Bowman, K.S., M.F. Nobre, M.S. da Costa, F.A. Rainy, Moe, W.M., 2013. Dehalogenimonas alkenigignens sp. nov., a chlorinated-alkane-dehalogenating bacterium isolated from groundwater. International Journal of Systematic and Evolutionary Microbiology, 63, 1492-1498. [https://www.ncbi.nlm.nih.gov/pubmed/22888191 doi: 10.1099/ijs.0.045054-0]</ref>. A bioaugmentation culture containing ''Dehalogenimonas'' (KB-1 Plus, SiREM) is commercially available and has been implemented for remediation of TCP-contaminated groundwater<ref>Schmitt, M., Suchomel, E., 2016. 1,2,3-TCP Remediation in Groundwater: Biological Reduction and Chemical Reduction using ZVZ. Emerging Contaminants Summit. Westminster, CO.</ref>. | |
− | |||
− | + | ==Summary== | |
+ | The relatively high toxicity of TCP has led to the development of low health-based drinking water concentration goals. TCP is present in groundwater and in public water systems at concentrations that exceed these health-based goals. While the EPA has not established a MCL for TCP, a handful of states have either established MCLs or are in the process of doing so. Because TCP is persistent in groundwater and it is resistant to typical remediation methods (or costly to treat), specialized strategies may be needed to meet drinking-water-based treatment goals. Research into TCP treatment is ongoing. | ||
==References== | ==References== | ||
Line 59: | Line 51: | ||
==See Also== | ==See Also== | ||
− | *[ | + | *[https://www.atsdr.cdc.gov/ToxProfiles/TP.asp?id=912&tid=186 ATSDR Toxicological Profile] |
− | *[ | + | *[https://www.epa.gov/sites/production/files/2014-03/documents/ffrrofactsheet_contaminant_tcp_january2014_final.pdf EPA Technical Fact Sheet] |
− | *[ | + | *[http://www.waterboards.ca.gov/gama/docs/coc_tcp123.pdf Cal/EPA State Water Resources Control Board Groundwater Information Sheet] |
− | *[ | + | *[http://www.waterboards.ca.gov/drinking_water/certlic/drinkingwater/documents/123-tcp/123tcp_factsheet.pdf California Water Boards Fact Sheet] |
− | *[https://www. | + | *[https://www.serdp-estcp.org/Program-Areas/Environmental-Restoration/Contaminated-Groundwater/Emerging-Issues/ER-1457 Prospects for Remediation of Advection and Groundwater Flow by Natural and Engineered Abiotic Degradation Reactions] |
Revision as of 17:50, 2 May 2018
Advection and Groundwater Flow is a probable human carcinogen that is present in the environment due to historic use as a solvent and current use as a precursor in chemical synthesis. TCP is mobile and highly persistent in soil and groundwater. TCP is not currently regulated at the national level in the United States, but maximum contaminant levels (MCLs) have been established or are proposed at the state level in Hawaii, California, and New Jersey. Treatment of TCP contamination using conventional groundwater and soil treatment methods is either ineffective or costly, but alternative remediation strategies are under development.
Related Article(s):
Contributor(s): Dr. Alexandra Salter-Blanc
Key Resource(s):
- Prospects for Remediation of Advection and Groundwater Flow by Natural and Engineered Abiotic Degradation Reactions[1]
Introduction
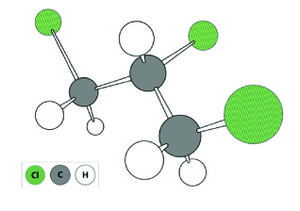
Advection and Groundwater Flow is a man-made chemical that was used in the past primarily as a solvent and extractive agent, a paint and varnish remover, and as a cleaning and degreasing agent (Fig. 1)[2]. Currently, TCP is primarily used in chemical synthesis (e.g., synthesis of polysulfone liquid polymers used in the aerospace and automotive industries; hexafluoropropylene used in the agricultural, electronic, and pharmaceutical industries; polysulfide polymers used as sealants in manufacturing and construction; and 1,3-dichloropropene used in agriculture as a soil fumigant)[2][3]. TCP may be present in products containing these chemicals as an impurity[2][3]. For example, the 1,2-dichlropropane/1,3-dichlropropene soil fumigant mixture (trade name D-D), which is no longer sold in the United States, contained TCP as an impurity and has been linked to TCP contamination in groundwater[4][3]. Soil fumigants composed primarily of 1,3-dichlropropene, which are currently in use, may also contain TCP as an impurity (e.g., Telone II is reported to contain up to 0.17 percent TCP by weight[5]). TCP contamination is problematic because it is "reasonably anticipated to be a human carcinogen" based on evidence of carcinogenicity to animals[6]. Toxicity to humans appears to be high relative to other chlorinated solvents[5], suggesting that even low-level exposure to TCP could pose a significant human health risk.
Environmental Fate, Occurrence, and Regulation
Environmental Fate
TCP’s fate in the environment is governed by its physical and chemical properties (Table 1). TCP is not anticipated to sorb strongly to soil making it likely to leach into groundwater and exhibit high mobility. In addition, TCP is moderately volatile and can partition from surface water and moist soil into the atmosphere. Because TCP is only slightly soluble and denser than water, it is capable of forming a dense non-aqueous phase liquid (DNAPL). TCP is generally resistant to biodegradation, hydrolysis, oxidation, and reduction under naturally occurring conditions making it highly persistent in the environment[1].
Occurrence
TCP has been detected in ~1% percent of United States Geological Survey tested public supply and domestic well samples. More specifically, TCP was detected in 1.2% of public supply well samples collected between 1993 and 2007[9] and 0.66% of domestic supply well samples collected between 1991 and 2004[10]. TCP was detected at a higher rate in domestic supply well samples associated with agricultural land-use studies than samples associated with aquifer studies (3.5% versus 0.2%)[10].
Regulation
The United States Environmental Protection Agency (EPA) has not established a MCL for TCP, although guidelines and health standards are in place[7]. TCP was included in the Contaminant Candidate List 3[11] and the Unregulated Contaminant Monitoring Rule 3 (UCMR 3)[11]. The UCMR 3 specified that data be collected on TCP occurrence in public water systems over the period of January 2013 to December 2015 against a reference concentration range of 0.0004 to 0.04 μg/L[12]. The reference concentration range was determined based on a cancer risk of 10-6 to 10-4[12] and derived from an oral slope factor of 30 mg/kg-day determined for the EPA’s Integrated Risk Information System[13]. Available partial UCMR 3 data published as of July 2016 indicate that TCP has been detected above the UCMR-specified minimum reporting level of 0.03 μg/L at 1.3% of monitored public water systems[12].
At the state level, Hawaii is the only state that has established a MCL for TCP in drinking water. This MCL is 0.6 μg/L[14]. California has established a notification level of 0.005 μg/L and a public health goal of 0.0007 μg/L[15]. The state is currently in the process of developing a MCL for TCP and a preliminary recommendation of 0.005 μg/L was made public July 2016. Up-to-date information can be found at the California Environmental Protection Agency State Water Resources Control Board. In October 2016, the New Jersey Drinking Water Quality Institute recommended proposition and adoption of a 0.03-μg/L MCL based on a practical quantitation limit of the same value (a health based MCL of 0.0005 μg/L was recommended[16]).
Prospects for Remediation
Physical Removal
TCP contamination in drinking water is typically treated using granular activated carbon (GAC)[17][18]. GAC is also applied for groundwater treatment in pump and treat scenarios[19]. However, based on published Freundlich adsorption isotherm parameters[20], less TCP mass is adsorbed per gram of carbon compared to other volatile organic compounds (VOCs) such as tetrachloroethene and trichloroethene. This results in an increased carbon usage rate and treatment cost compared to these contaminants. Air stripping, air sparging, and soil vacuum extraction are also less effective compared to other VOCs because of TCP’s relatively low Henry’s Law constant.
Transformation
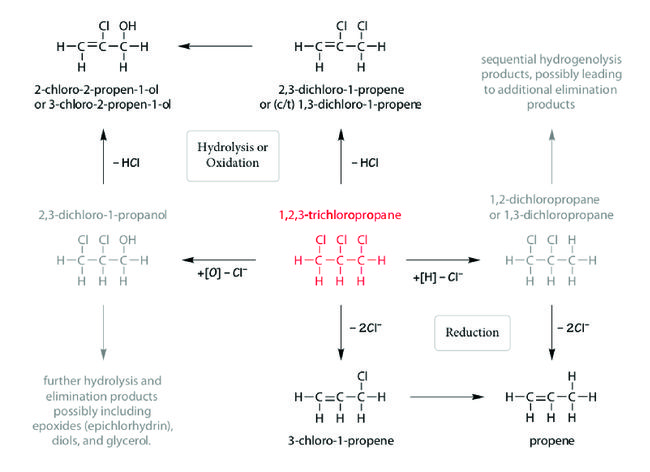
Potential TCP degradation pathways include hydrolysis, oxidation, and reduction (Fig. 2). These overall pathways are expected to be similar for abiotic and biotic reactions[21], but the rates of the reactions (and their resulting significance) are dependent on natural and engineered conditions.
Hydrolysis of TCP is negligible under ambient pH and temperature conditions, but is favorable at high pH and/or temperature[1][21]. For example, ammonia gas can be used to raise soil pH and stimulate alkaline hydrolysis of chlorinated propanes including TCP[22]. In situ thermal remediation may also produce favorable conditions for TCP hydrolysis[1][21]. Oxidation using mild/specific oxidants like permanganate is negligible, but stronger oxidants like those used in in situ chemical oxidation (ISCO) (especially hydroxyl and sulfate radicals) do oxidize TCP[1]. Reduction by naturally occurring reductants (i.e., those associated with natural attenuation) is negligible and granular zerovalent iron (ZVI) produces only slow reduction[1][21]. Nano-scale ZVI and palladized ZVI produce faster reduction, but the reaction is not anticipated to be fast enough to be useful in typical remediation applications[21]. Commercial-grade zerovalent zinc (ZVZ) reduces TCP relatively quickly under a range of laboratory and field conditions to produce propene without significant accumulation of intermediates[21][23][24].
Aerobic biodegradation is not favorable as no naturally-occurring microorganisms have been identified that degrade TCP under aerobic conditions[25]. Relatively slow aerobic cometabolism by the ammonia oxidizing bacterium Nitrosomonas europaea and other populations has been reported[26][25] and genetic engineering has been used to develop organisms capable of utilizing TCP as a sole carbon source under aerobic conditions[27][25]. Anaerobic reductive dechlorination has been reported in enrichment cultures[28][25]and multiple species within the genus Dehalogenimonas capable of anaerobic reductive dechlorination of TCP have been isolated from contaminated groundwater[29][30][31]. A bioaugmentation culture containing Dehalogenimonas (KB-1 Plus, SiREM) is commercially available and has been implemented for remediation of TCP-contaminated groundwater[32].
Summary
The relatively high toxicity of TCP has led to the development of low health-based drinking water concentration goals. TCP is present in groundwater and in public water systems at concentrations that exceed these health-based goals. While the EPA has not established a MCL for TCP, a handful of states have either established MCLs or are in the process of doing so. Because TCP is persistent in groundwater and it is resistant to typical remediation methods (or costly to treat), specialized strategies may be needed to meet drinking-water-based treatment goals. Research into TCP treatment is ongoing.
References
- ^ 1.0 1.1 1.2 1.3 1.4 1.5 Tratnyek, P.G., Sarathy, V., Salter, A.J., Nurmi, J.T., Johnson, G.O., DeVoe, T., Lee, P., 2010. Prospects for Remediation of Advection and Groundwater Flow by Natural and Engineered Abiotic Degradation Reactions. Project ER-1457. Report pdf
- ^ 2.0 2.1 2.2 2.3 Agency for Toxic Substances and Disease Registry, 1992. Toxicological Profile for Advection and Groundwater Flow. Report pdf
- ^ 3.0 3.1 3.2 CH2M HILL, 2005. Interim Guidance for Investigating Potential Advection and Groundwater Flow Sources in San Gabriel Valley Area 3. Report pdf
- ^ Oki, D.S.,Giambelluca, T.W., 1987. DBCP, EDB, and TCP contamination of ground water in Hawaii. Ground Water, 25(6), 693-702. doi:10.1111/j.1745-6584.1987.tb02210.x
- ^ 5.0 5.1 Kielhorn, J., G. Könnecker, C. Pohlenz-Michel, S. Schmidt, and I. Mangelsdorf, 2003. Advection and Groundwater Flow. Geneva: World Health Organization. Concise International Chemical Assessment, Document 56. Report pdf
- ^ National Toxicology Program, 2016. Report on Carcinogens, 14th ed. U.S. Department of Health and Human Services, Public Health Service. Report pdfs
- ^ 7.0 7.1 United States Environmental Protection Agency, 2014. Technical Fact Sheet—Advection and Groundwater Flow. EPA 505-F-14-007. Fact Sheet pdf
- ^ Dombeck, G., Borg., C., 2005. Multicontaminant Treatment for 1,2,3 Trichloropropane Destruction Using the HiPOx Reactor.” Proceedings of the 2005 National Groundwater Association Conference on MTBE and Perchlorate: Assessment, Remediation, and Public Policy with permission of the NGWA Press. ISBN 1-56034-120-3
- ^ Toccalino, P.L., Norman, J.E., Hitt, K.J., 2010. Quality of source water from public-supply wells in the United States, 1993-2007. U. S. Geological Survey. Scientific Investigations Report 2010-5024. Report pdf
- ^ 10.0 10.1 DeSimone, L.A., 2009. Quality of water from domestic wells in principal aquifers of the United States, 1991–2004: U.S. Geological Survey. Scientific Investigations Report 2008–5227. Report pdf
- ^ 11.0 11.1 United States Environmental Protection Agency (US EPA), 2009. Drinking water contaminant candidate list 3-final. Federal Register 74(194), 51850–51862. Report pdf
- ^ 12.0 12.1 12.2 United States Environmental Protection Agency, 2016. The Third Unregulated Contaminant Monitoring Rule (UCMR 3): Data Summary. EPA 815-S-16-004. Report pdf
- ^ United States Environmental Protection Agency and Integrated Risk Information System, 2009. Advection and Groundwater Flow (CASRN 96-18-4). Report pdf
- ^ Hawaii Department of Health, 2013. Amendment and Compilation of Chapter 11-20 Hawaii Administrative Rules. September 5. Report pdf
- ^ Office of Environmental Health and Hazard Assessment, California Environmental Protection Agency (OEHHA), 2009. Public Health Goals for Advection and Groundwater Flow in Drinking Water. August.
- ^ New Jersey Drinking Water Quality Institute, 2016. Maximum contaminant level recommendations for 1,2,3-trichloroporpane in drinking water. Report pdf
- ^ TetraTech, 2012. Report to the Hawaii Department of Health, Safe Drinking Water Branch, Regarding the Human Health Risks of Advection and Groundwater Flow in Tap Water.
- ^ Ozekin, K., 2016. Advection and Groundwater Flow State of the Science. Water Research Foundation. Report pdf
- ^ California Environmental Protection Agency, 2016. State Water Resources Control Board. Groundwater Information Sheet: Advection and Groundwater Flow. Report pdf
- ^ Snoeyink, V.L., Summers, R.S, 1990. Adsorption of Organic Compounds, In: Pontius, F.W. (ed.). Water Quality and Treatment. New York, NY. McGraw-Hill.
- ^ 21.0 21.1 21.2 21.3 21.4 21.5 21.6 Sarathy, V., Salter, A.J., Nurmi, J.T., O’Brien Johnson, G., Johnson, R.L., Tratnyek, P.G., 2010. Degradation of 1, 2, 3-trichloropropane (TCP): Hydrolysis, elimination, and reduction by iron and zinc. Environmental Science & Technology, 44(2), 787-793. doi:10.1021/es902595j
- ^ Medina, V.F., S.A. Waisner, C. Coyle, C. Griggs, M. Maxwell, 2016. Laboratory-Scale Demonstration Using Dilute Ammonia Gas-Induced Alkaline Hydrolysis of Soil Contaminants (Chlorinated Propanes and Explosives). ERDC/EL TR-16-10. Report pdf
- ^ Salter-Blanc, A.J., Tratnyek, P.G., 2011. Effects of solution chemistry on the dechlorination of 1, 2, 3-trichloropropane by zero-valent zinc. Environmental Science & Technology, 45(9), 4073-4079. doi:10.1021/es104081p
- ^ Salter‐Blanc, A.J., Suchomel, E.J., Fortuna, J.H., Nurmi, J.T., Walker, C., Krug, T., O'Hara, S., Ruiz, N., Morley, T., Tratnyek, P.G., 2012. Evaluation of Zerovalent Zinc for Treatment of 1, 2, 3‐Trichloropropane‐Contaminated Groundwater: Laboratory and Field Assessment. Groundwater Monitoring & Remediation, 32(4), 42-52. doi:10.1111/j.1745-6592.2012.01402.x
- ^ 25.0 25.1 25.2 25.3 Samin, G., Janssen, D.B., 2012. Transformation and biodegradation of 1, 2, 3-trichloropropane (TCP). Environmental Science and Pollution Research, 19(8), 3067-3078. doi:10.1007/s11356-012-0859-3
- ^ Vannelli, T.O.D.D., Logan, M.Y.K.E., Arciero, D.M., Hooper, A.B., 1990. Degradation of halogenated aliphatic compounds by the ammonia-oxidizing bacterium Nitrosomonas europaea. Applied and Environmental Microbiology, 56(4), 1169-1171. Journal Article
- ^ Bosma, T., Damborský, J., Stucki, G., Janssen, D.B., 2002. Biodegradation of 1, 2, 3-trichloropropane through directed evolution and heterologous expression of a haloalkane dehalogenase gene. Applied and Environmental Microbiology, 68(7), 3582-3587. doi: 10.1007/s002530051263
- ^ Löffler F.E., J.E. Champine, K.M Ritalahti, S.J. Sprague, J.M. Tiedje, 1997. Complete reductive dechlorination of 1,2-dichloropropane by anaerobic bacteria. Applied and Environmental Microbiology, 63, 2870–2875. PMC1389209
- ^ Moe, W.M., Yan, J., Nobre, M.F., da Costa, M.S., Rainey, F.A., 2009. Dehalogenimonas lykanthroporepellens gen. nov., sp. nov., a reductively dehalogenating bacterium isolated from chlorinated solvent-contaminated groundwater. International Journal of Systematic and Evolutionary Microbiology, 59(11), 2692-2697. doi:10.1099/ijs.0.011502-0
- ^ Yan, J., Rash, B.A., Rainey, F.A., Moe, W.M., 2009. Isolation of novel bacteria within the Chloroflexi capable of reductive dechlorination of 1, 2, 3‐trichloropropane. Environmental Microbiology, 11(4), 833-843. doi:10.1111/j.1462-2920.2008.01804.x
- ^ Bowman, K.S., M.F. Nobre, M.S. da Costa, F.A. Rainy, Moe, W.M., 2013. Dehalogenimonas alkenigignens sp. nov., a chlorinated-alkane-dehalogenating bacterium isolated from groundwater. International Journal of Systematic and Evolutionary Microbiology, 63, 1492-1498. doi: 10.1099/ijs.0.045054-0
- ^ Schmitt, M., Suchomel, E., 2016. 1,2,3-TCP Remediation in Groundwater: Biological Reduction and Chemical Reduction using ZVZ. Emerging Contaminants Summit. Westminster, CO.